
A faster and more efficient new brain-imaging technique is resulting in the ability to characterise the brain’s tissue properties with a high-quality image resolution. The team from University of Queensland (UQ) Centre for Advanced Imaging (CAI) are now able to map the magnetic tissue properties of the whole brain in under 40 seconds, which is seven times faster than common techniques that have the same image quality – and is achieved by combining advanced data acquisition with novel image reconstruction techniques.
Medical Imaging Technology: Can you tell us about the research and its purpose?
Dr Daniel Stäb: Our research employs a novel, fast MRI sequence at the ultra-high-field strength of 7T to map the susceptibility of brain tissue, a parameter that contains information on iron and myelin, which are important components, or brain metabolism. Mapping magnetic susceptibility is potentially valuable for the diagnosis of neurodegenerative diseases, such as Alzheimer’s, Parkinson’s, Huntington’s disease, or multiple sclerosis.
The very high magnetic field strength provides us with much higher signal and sensitivity than conventional clinical MRI scanners. The novel image acquisition method that I have developed together with my colleagues, Dr Steffen Bollmann and Associate Professor Markus Barth, translates this signal-to-noise advantage into the very high spatial resolution that is necessary to resolve tiny brain structures relevant for memory and loss of memory in neurodegenerative diseases; for example, substructures and layers of the hippocampus.
How does the new brain-mapping technique differ from the normal way – particularly with regard to the detail and scope of options it provides?
In MRI, Excite spins and obtains a signal while the spins relax back to the equilibrium state. The relatively new method of magnetic-susceptibility mapping in the brain is usually performed using a 3D gradient-echo sequence, where this process needs to be repeated for every single line of a 3D image. Having sampled every line, a full 3D image volume is calculated/reconstructed.
As the sampling process takes a certain amount of time for each image line, and for high spatial resolutions, a large number of repetitions is required; the acquisition times are typically very long – five to ten minutes.
To significantly reduce this acquisition time, we use an echo-planar-imaging (EPI) sequence with a planes-on-a-paddlewheel (POP) readout scheme. Sampling multiple lines or whole 2D planes of an image for each excitation, the sequence is extremely scan-time efficient and much faster than the conventional approach. As such, we were able to reduce the acquisition time from about five minutes to 40 seconds. In addition, the advanced sampling scheme provides some inherent stability with respect to subject motion, which is important when considering patients
in pain, or elderly patients.
How well do you really know your competitors?
Access the most comprehensive Company Profiles on the market, powered by GlobalData. Save hours of research. Gain competitive edge.
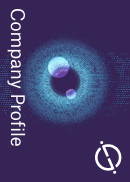
Thank you!
Your download email will arrive shortly
Not ready to buy yet? Download a free sample
We are confident about the unique quality of our Company Profiles. However, we want you to make the most beneficial decision for your business, so we offer a free sample that you can download by submitting the below form
By GlobalDataThe technique was used by combining ‘an advanced data acquisition with novel image reconstruction techniques’; how does the data acquisition help and can you explain this in more detail?
Conventional EPI is a Cartesian imaging technique widely used in clinical routine. It is very scan-time efficient, but comes with some adverse effects such as dropout artefacts or geometric distortions. These adverse effects can get severe at high fields. Moreover, at high spatial resolutions, we experience limitations in sequence timing.
To resolve these timing issues and, at the same time, reduce the aforementioned adverse effects, we propose to use a so-called non-Cartesian POP readout scheme. With this advanced sampling scheme, the 3D sampling space is not rectangular but cylindrical, and is not sampled slice or partition-wise but as planes/paddles on a paddlewheel.
We were able to tailor the data acquisition to the actual shape of the brain, which helps in reducing dropout artefacts and effectively suppresses geometric image distortions within axial views.
In addition, the non-Cartesian sampling scheme allows for further improvements, and optimisations of WRT speed and spatial resolution during data acquisition, and provides us with some inherent stability with regard to subject motion.
Early detection for neurodegenerative diseases is very important; why is this, and how exactly will these mapping techniques help?
Many neurodegenerative diseases such as Parkinson’s and Alzheimer’s are basically the consequence of the loss of structure or function of neurons; for example, the brain cells that constitute the fundamental building blocks of the brain. The underlying processes that drive the neurodegeneration are very complex and not yet fully understood. Research aims to unravel these processes and to develop effective treatments for the different neurodegenerative diseases. In this context, one goal is to identify suitable biomarkers for early disease detection.
Recent studies indicate that measurements of the magnetic bulk susceptibility can reflect changes of the iron and myelin content, and distribution in brain tissue. Thus, mapping the magnetic susceptibility is potentially of value for the detection of disease-related tissue changes in the human brain.
It is noteworthy that susceptibility mapping also has a number of other applications, including the visualisation of microbleeds, the potential to investigate iron storage and the visualisation of deep-brain nuclei to aid electrode planning for deep-brain stimulation.
What, if any, specific goals did the research have?
The main goal of our research was to develop a flexible and fast approach for high-resolution susceptibility mapping of the brain that would facilitate its application in the clinical field and elderly patients. The conventional spoiled gradient-echo approach is rather slow, and mapping the brain with high spatial resolution can take very long. Apart from being uncomfortable for the patient, the likelihood of head motion during a scan increases with the scan time. As subject motion can significantly impair the image quality, novel and faster methods are required to effectively translate these brain-mapping techniques into clinical applications.
Are there any other specific benefits this can provide, such as quicker treatments or a higher level of patient care, or monetary or space savings?
First of all, faster scans directly reduce the time a patient has to lie inside the MRI scanner. Apart from increasing the patient’s comfort, this means that more patients can be examined in the same time, which can reduce scan costs.
However, subject motion is currently one of the limiting factors in QSM and often prevents us from conducting very long measurements that would be required to achieve very high spatial resolutions.
With the increase in imaging speed and the signal-to-noise benefit from scanning at ultra-high magnetic fields, submillimetre spatial resolutions will become feasible.
What are the plans for the future of this type of research and the advances in technology it can provide?
So far, we could show that susceptibility mapping of the brain is feasible using our POP EPI sequence, and the results are comparable to the standard approach. Given that very promising start, we will include further subjects to detail the performance of our technique.
In addition to that, we will of course conduct further developments to fully exploit the benefits of the POP readout scheme; for example, the motion robustness. Importantly, we are actively pursuing to make this technique available to other researchers and, in a next step, even for clinical scanners to be used in patient examinations.
We are currently investigating further reductions of scan time and increased spatial resolutions. This will then hopefully provide means to delineate the smaller brain structures and advance the search for early disease biomarkers. Besides this, we consider applying our technique to other fields of brain MRI such as functional brain imaging.
What will you and the team work on next for medical imaging – where do you see the advances in MRI heading?
Tissue characterisation is certainly a hot topic in MRI at the moment – in fact, it has been a goal of researchers for many years. In analogy to pathology, which of course works only with extracted or diseased tissue, we want to use the MRI system to derive quantitative tissue properties in vivo. Currently, MRI provides images that have a certain MR parameter ‘weighting’, but it would be much more valuable to base a diagnosis on tissue parameters derived from quantitative MR measures.
The technical developments in the past decade have made huge steps in WRT accuracy in a new generation of MRI systems. Quantitative susceptibility mapping is only one part and a combination with the wider known MR parameters,
such as T1, T2 and diffusion.
Our multidisciplinary team is therefore investigating the combination of these parameters, and novel signal and imaging processing approaches with the aim of improving the accuracy of in-vivo tissue characterisation using MRI and ultimately improving diagnosis to help in treatment decisions.